PhD Research Report
Range uncertainty reduction in proton beam therapy via prompt gamma-ray detection - PDF Version
Abstract
Compared with conventional radiotherapy, proton therapy offers dosimetric advantages. Full exploitation of proton therapy, however, is hindered by the uncertainty in the fall-off positions of proton beams, or range uncertainty. A new method, called prompt gamma coincidence (PGC), has been developed to monitor the proton range in tissues through the detection of 2.741/6.128 MeV 16O-induced prompt gamma rays that are emitted by coincidence. Prompt gamma rays are gamma rays that are emitted naturally from nuclear de-excitations after proton bombardment.
The detection system comprises 16 cerium-activated lanthanum bromide (LaBr3(Ce)) detectors. The information that is recorded by the system is fed into a reconstruction algorithm. Two versions of the algorithm, Algorithm 1 and 2, have been proposed. They are based on different input data and 3D geometry.
In the first study, the system was simulated with a water phantom inside its volume. A 180MeV proton beam was shot on to the phantom, along its central axis. The simulation output was imported into Algorithm 1. To investigate different clinical scenarios, the system’s internal radius was set at 8cm, 15cm and 25cm. A 5mm and 10mm range undershoot was also modelled.
Ιn a second/third study, the emission position reconstruction was reported for an isotropic point cobalt (60Co) source. The evaluation was both in-silico and experimental. The experimental validation was performed at the National Physical Laboratory, with only eight LaBr3(Ce) detectors in place. The internal volume radius was always 9cm. The output of all evaluations was processed by Algorithm 2. All simulations were performed using the Monte Carlo-based Geant4 (10.04) toolkit.
Using Algorithm 1, the peak in the prompt gamma profile, for a 180MeV beam that was shot on to a water phantom, was inferred with a sigma of 4.17mm, 5.65mm, and 6.36mm for an 8cm, 15cm, and 25cm internal radius. For an 8cm radius, with a 5mm and 10mm undershoot, the sigma was 4.31mm and 5.47mm. These values are comparable with the range uncertainties that are incorporated into treatment planning.
Using Algorithm 2, simulation results showed that the emission position of a 60Co source at (0,0,0) was reconstructed within 3mm and 1mm for 105 and 106 primary events, respectively. Experimental results showed that for sources at (0,0,0) and (0,0,-30) mm, with 106 recorded events, the emission position was reconstructed within 5mm.
Work is being carried out to optimise further the detection system and the algorithms. In light of the positive tests, the first PGC prototype is being constructed. Experimental validation with clinical proton beams is planned.
Which institution were you affiliated to during your PhD?
University of Manchester, Division of Cancer Sciences.
What was the motivation for the topic of your PhD work?
The full exploitation of proton therapy is hindered by the range uncertainty. The sources of range uncertainty are numerous, even if only non-moving targets are considered. Range uncertainty is managed clinically by making plans robust against uncertainties. The way this is achieved depends on the treatment planning approach. In single field, uniform dose (SFUD) plans, a distal expansion of the clinical target volume (CTV) ensures that there is coverage despite range uncertainty. Conversely, in intensity modulated proton therapy (IMPT), robust optimisation incorporates range and set-up uncertainties into the treatment plan.
Whatever approach is chosen, there is always the need to prove its success by means of in-vivo range verification. The most promising range verification method is via prompt gamma detection. Prompt gamma (PG) rays are gamma rays that are emitted naturally after nuclear inelastic reactions between protons of the beams and isotopes of oxygen, carbon and nitrogen. However, the development of a clinically usable prompt gamma ray detection system has progressed slowly. This is mainly because the energy of the rays is too high to be registered by the standard devices that are employed in diagnostic imaging.
What were the main findings of your PhD?
In the framework of my PhD project, a new method, called prompt gamma coincidence (PGC), was developed. The PGC method reconstructs the proton range in 3D via prompt gamma detection. The PGC method, in particular, focuses only on the 2.741MeV and 6.128MeV 16O-induced PG lines. These gamma lines are emitted in cascade. The time difference between the two lines is negligible, so they can be regarded as simultaneously emitted in time and position. During proton-beam irradiation, several 2.741MeV and 6.128MeV prompt gamma couples are produced. By detecting these couples, the emission positions can be reconstructed. Eventually, from all the emission positions, the range is inferred. The PGC method is based on two components. These components are: the detection system, to record the 16O-induced PG couples; and the reconstruction algorithm, to retrieve the couple emission positions.
Can you comment on the impact of your work to the field?
Ι have developed a new PG detection system, which could be employed in the clinical environment to assess in-vivo range verification. The system does not have any mechanical collimation. Usually, PG detection systems are severely affected by neutron-induced background noise. High-energy neutrons are produced along the beam line and in phantoms. In the PGC method, however, the requirement for coincidences rejects the neutron-induced gamma background. For all these reasons, I consider that the new concept that is proposed by the PGC method is advantageous for in-vivo range verification as well as for proton therapy quality assurance procedures.
What was the most challenging part of your PhD?
I had to focus on both the design of the PG spectrometer and the conceptualisation and coding of the reconstruction algorithm. Although different, these two components were connected in such a way that any modification in one deeply affected the other. In particular, the conceptualisation of specific mathematical steps in the algorithm was extremely challenging.
Who or what inspired you most during your studies?
My family and my friends have always been sources of strength and support. A great inspiration came also from Marios, my PhD buddy, best friend and partner (in chronological order). The full thesis was written during home-working during the Covid-19 pandemic. I owe a debt of gratitude to all the essential workers and healthcare personnel who have been working through this difficult year.
When did you defend your thesis and who was your supervisor?
I defended my thesis on Friday, 13 November. My main supervisor was Doctor Michael Taylor, my co-supervisor was Professor Ranald Mackay.
Will you stay in the field? What are your plans for the future?
Yes, I will stay in the field. I have accepted a position as research fellow in the radiation oncology department of the Mayo Clinic, Rochester, Minnesota (USA).
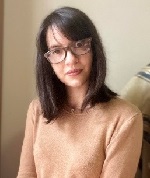
Costanza Panaino
Previously at:
Division of Cancer Sciences
University of Manchester
Manchester, U.K.
About the author
Costanza Panaino received pre-university education at Ravenna, Italy, where she obtained her diploma in 2010. In the same year, she started her studies at the University of Milano-Bicocca, where she received her BSc degree in physics in 2013. For her bachelor's thesis, she conducted a research study on Geant4 simulation of X-ray tubes for medical diagnostics. She continued her graduate studies at the University of Milan, where she was awarded a MSc degree in physics in 2016, with elective courses in medical and nuclear physics. During 2015 and 2016, she spent 13 months within the Department of Medical Physics at the National Cancer Institute of Milan, to research prostate intra-fraction motion during conventional radiotherapy, which was the topic of her master's thesis. From 2016 to 2020, she was enrolled in a PhD project at the University of Manchester but was based at the Christie NHS Foundation Trust. She pursued an investigation on range uncertainty reduction in proton-beam therapy, which is the subject of her PhD thesis. She presented her work in two peer-reviewed publications and at several international conferences. At the 2019 annual conference of the Particle Therapy Co-Operative Group (PTCOG), she was selected as a finalist in the young investigator award